
By constricting the pool of available mRNA templates, RNAi decreases the amount of proteins built from those mRNAs. In RNAi, a short RNA threads into a protein called Argonaute, and the RNA-Argonaute complex then pairs with an mRNA segment that matches the RNA’s sequence. To exert their effects, miRNAs act as guides in a gene-silencing pathway related to RNA interference (RNAi), a mechanism found in most organisms that protects the cell from invaders, such as viruses or transposons. In many cases the role of a miRNA is so important that removing its activity in mouse studies can have dramatic effects, including death (typically before birth or soon after birth), epilepsy, deafness, infertility, and cancer. More often, miRNAs optimize gene expression, customizing it for many genes in each cell type. Whitehead Institute Member David Bartel likens miRNAs to sculptors of the transcriptome, chiseling away at gene expression to such an extent that in some cases miRNAs can trigger developmental changes.
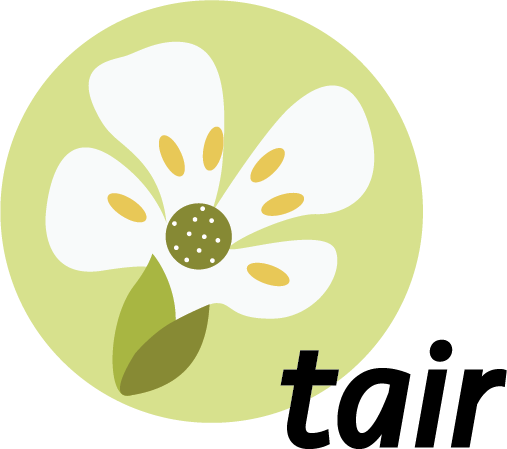
MiRNAs: Redefining gene regulation and spawning a new avenue for therapeuticsĪt only 22 base pairs long, miRNAs may be small, but their effects resonate throughout evolution and development in both plants and animals. Their foundational work has transformed researchers’ understanding of gene regulation and revealed a complex, elegant, and highly responsive system that tailors gene expression for each cell type and tissue. By folding into different shapes, certain mRNAs can alter their fate to produce templates for two, three, even one hundred different proteins.įrom their work characterizing some of the first miRNAs and lncRNAs, to connecting RNA form to function, Whitehead Institute scientists have been at the forefront of discovering the many ways that RNAs can regulate gene expression. In some cases mRNAs can even influence the proteins created from them. Modifying protein production is not limited to noncoding RNAs. Tools and methods developed over the past thirty years have revealed within this “junk DNA” a panoply of critical elements that include sequences encoding regulatory RNAs, such as microRNAs (miRNAs) that tune protein production and long noncoding RNAs (lncRNAs), some of which can also alter gene transcription. The DNA sequences that encode most regulatory RNAs reside in what researchers once dismissed as genomic dead weight - the more than 97% of the human genome that is considered “noncoding” DNA because it does not produce mRNA templates for proteins. Collectively their research shows that frequently it is regulatory RNAs’ influence that determines whether or how a gene’s DNA sequence ends up as a viable protein. Scientists at Whitehead Institute and around the world have uncovered hundreds of these “regulatory RNAs” and many more RNAs that might also have regulatory functions. Added into this mix are different types of RNAs that attenuate or enhance gene expression. Some proteins effectively barricade certain genes, blocking access and making them difficult to transcribe, while other proteins can remodel the final protein products.
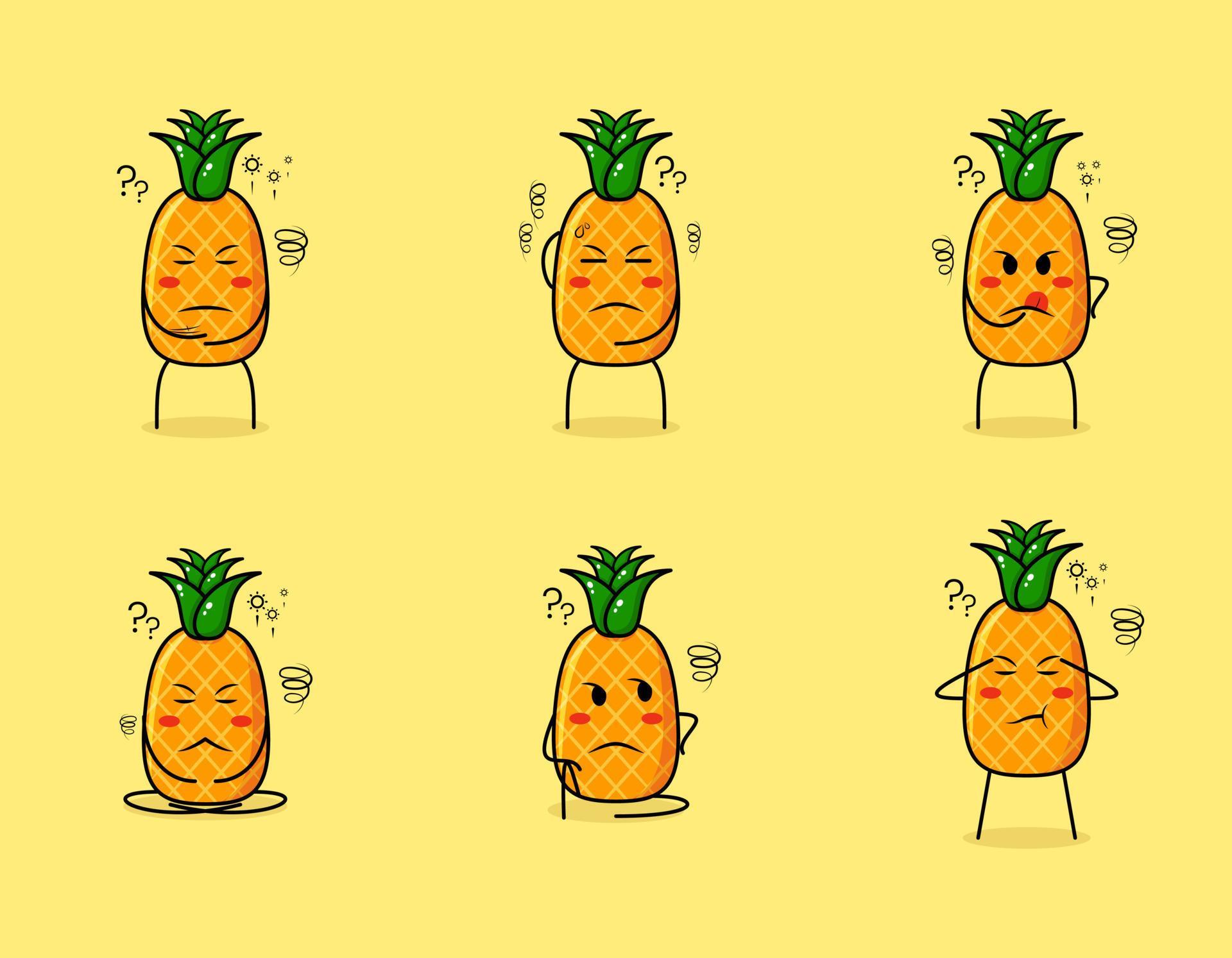
But as scientists have been able to explore more deeply how the DNA sequence of our genes are read or “transcribed” into RNAs called messenger RNAs (mRNAs), and those mRNAs are ultimately translated into proteins, the mechanisms regulating gene expression have gotten, well, complicated. In the late 1950s, Francis Crick proposed his “central dogma”: DNA begets RNA, RNA begets protein, and protein cannot transfer information back to DNA or RNA. Click here to see all stories in this collection. This story is part of our series, Looking Beyond the Gene.
